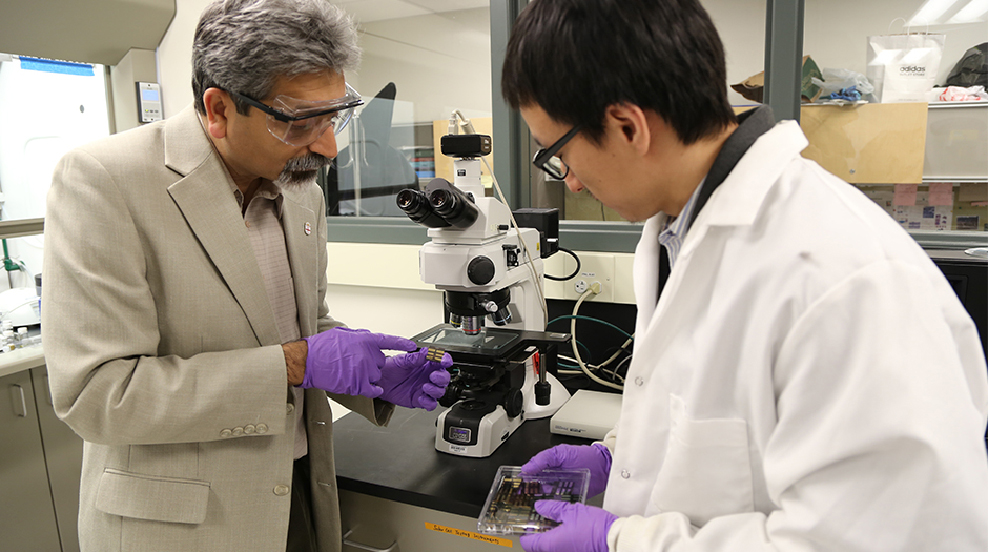
Researchers in Brown's School of Engineering are developing next-generation renewable energy technologies, advancing energy efficiency in computing and finding new ways to detect and clean contaminants in the environment.
As global temperatures rise, economies expand and population grows, the need increases for new environmental and energy technologies to create a cleaner, more sustainable future for the planet. In the lab and in the field, researchers from Brown University's School of Engineering are working collaboratively to develop the next generation of green technologies to meet these generational challenges.
"Over the last 150 years, the global economy became incredibly sophisticated technologically, and this has enabled us to create a lot of indispensable things. But we've done it by polluting the air and water and dumping over a thousand billion tons of carbon into the atmosphere," said School of Engineering Dean Larry Larson. "If we're going to build a cleaner post-carbon world, we're going to need new energy sources, more efficient devices, new manufacturing techniques and new ways of cleaning up after ourselves. Engineering research is going to be at the very center of all those efforts, and addressing these multifaceted challenges is the kind of research that thrives here at Brown."
As the University commits itself to net-zero emissions by 2050, researchers from Brown are creating new ways of harvesting energy from the sun, while other teams develop new batteries for storing that energy. Computer engineers are devising systems that use power more efficiently in a world increasingly reliant on mass computation. And teams of scholars are developing new ways of identifying and eliminating environmental pollutants.
Like many emerging areas of research in engineering, Larson said, these programs tend not to fit well into traditional disciplinary structures. Brown's engineering school has no departmental constructs separating disciplines, which encourages new collaborations and ensures research can be pursued across boundaries.
"When you think about making batteries, for example, you're talking about something that involves chemistry, material science, mechanics and more," Larson said. "At Brown, you can walk down the hall and talk to experts in any of these areas — whoever you need. That's harder to do in a place where each one of those disciplines is in a separate building. That's one reason why Brown is so strong in these research areas."
The most recent major investment in engineering at Brown, the new Engineering Research Center provides the state-of-the-art facilities this kind of research demands, including a 4,000 square-foot clean room for nanotechnology and electronics research, a new electron microscope facility and open lab spaces designed to encourage the kind of collaborative research that thrives at Brown.
And among the researchers making use of that new building are students, who work directly alongside accomplished faculty. Through a recently created undergraduate concentration in environmental engineering, as well as significant student participation in research by undergraduates and graduate students alike, Brown is developing the top energy and environmental engineers of tomorrow.
Solar Cells and Batteries
Over the past 10 years, solar cells made from crystalline materials known as perovskites have emerged as a potential next-generation solar technology. Perovskites cost a fraction of traditional silicon, but are just as efficient in converting sunlight to energy. And perovskite solar cells can be made from thin, semi-transparent films, raising the possibility of energy-generating windows and other building-integrated solar technologies. They may also be a great option for small, flexible devices — solar cells on backpacks that can charge a cell phone, for example.
Supported by a $4 million grant from the National Science Foundation and funding from the Office of Naval Research, engineering professor Nitin Padture is working to overcome remaining challenges to adoption of this technology — making perovskites safer, more durable and commercially viable.
One issue is that perovskites generally contain lead, which is toxic, as well as organic materials, which degrade quickly when exposed to the environment. Padture's lab is leading efforts to make lead-free, inorganic perovskites. The team's research has revealed several material options, one made with titanium and another with tin, that eliminate lead and organics while maintaining reasonable solar efficiency.
Padture's group has also made strides in developing new manufacturing techniques for perovskites, which could help in speeding them to market. They have demonstrated several methods for making cells large enough for commercial applications while retaining the efficiency of smaller, lab-scale cells. Most recently, the group has worked to improve durability.
"I think the major promise of perovskites is in bringing solar into places where it hasn't been widely used before — tents and backpacks and all kinds of consumer products," Padture says. "But if we're going to have these cells where people interact with them, we have to make them lead-free, stable and durable. If we can do that, then we can really see the promise of these materials realized."
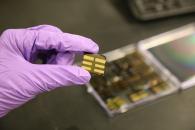
While Padture's lab works on new ways of harvesting energy, fellow faculty member Brian Sheldon and colleagues are working on new ways of storing it. With support from the National Science Foundation, General Motors and the U.S. Department of Energy, Sheldon is developing new strategies for improving lithium ion batteries, the rechargeable batteries used in everything from cell phones to electric cars. In addition to reducing fossil fuel use in transportation, new battery technologies will make better use of renewable energy sources like wind and solar, storing power for when sun and wind energy is not available.
"The traditional battery research community has largely been electrochemists," Sheldon said. "But there are a lot of problems in energy storage where mechanical issues are critical, and one of Brown's strengths has been in the mechanical behavior of materials. So our focus has been on bringing that mechanics and materials expertise to energy storage problems."
One area where mechanical insights have had a major impact is in understanding solid-electrolyte interphases (SEIs) — a film that forms between a battery's anode and its electrolyte. SEIs are critical to battery function, and finding ways of strengthening them will enable batteries with vastly longer lifetimes.
Sheldon's lab developed a way to test the mechanical strain on SEIs in actual battery conditions — something no one had been able to do previously. Using atomic force microscopy, the team was able to investigate the failure mechanisms of SEIs for the first time.
Now he is bringing his mechanics expertise to the study of solid electrolytes. In most current batteries, the electrolyte is liquid. That works fine, but defects can lead to fires — remember those exploding mobile phones reported nationwide last year? Solid electrolytes are potentially more stable, but understanding the strains placed on them during charging and discharging cycles is critical. Recent research by Sheldon and colleagues is starting to shed light on the factors that lead to solid electrolyte failure. These observations will be key in improving the design of solid electrolytes.
Sheldon's insights into battery technology were possible, he says, because of the diversity of expertise at Brown.
"We and a few other folks have pushed the idea that mechanics are important in batteries, and I think we can take some credit for being part of the first wave of this," he said. "One of the nice things about Brown is we have a lot of interdisciplinary expertise — partly because of the way we're constructed. We don't have traditional departmental boundaries so people's research interests here are interwoven."
Energy-Efficient Computing
From the devices that we hold in our hands to the warehouses full of servers that store and manipulate the world's data, computing generates an ever-increasing demand for energy.
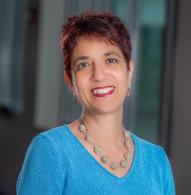
"Individually, our devices don't consume a lot of power, but cumulatively they do," said Iris Bahar, Professor of Engineering and Computer Science. "And you also have these big data centers used by Google and others that collectively require the equivalent of several power plants just to run them."
To save energy and make more reliable devices, Bahar and other computer engineers at Brown are working to develop computing systems that use energy more efficiently. The key to doing that, Bahar said, is to approach the problem at both the hardware and software levels. For software, that means writing algorithms that use a computer's hardware resources more efficiently. The hardware needs to be designed to make smart use of power.
One approach they have developed is a joint hardware-software framework called IgnoreTM. The framework enables computing systems to selectively ignore the errors that arise when the system is running at lower voltages without adversely compromising accuracy. In testing of the framework, Bahar and colleagues showed that it enabled systems to use 47% less energy with minimal impact on performance.
Sherief Reda, a professor of engineering, has done extensive work supported by the National Science Foundation to devise new methods to enable large data centers to work efficiently under power caps. Global data centers consume about 3% of total electricity consumption worldwide. The amount of power available on the power grid varies significantly throughout the day, and grid operators have programs to manage these power peaks and valleys. For data centers, that sometimes means capping the total amount of power they consume. Reda's lab developed a system called PowerCOORD that uses machine learning to optimize the servers to run under power caps, potentially helping data centers to use less power while meeting their performance requirements. A paper describing PowerCOORD won a best paper award at the 2018 IEEE International Green and Sustainable Computing Conference.
Both Bahar's and Reda's efforts are new strategies for limiting the amount of power that our computing technologies require, which is critical at a time when the majority of the world's power is still derived from carbon-emitting fossil fuels.
Superfund Research Program
Nanotechnology has revolutionized modern engineering. Working at the tiniest scales, researchers are designing new materials and devices with amazing properties. In particular, ultra-thin materials like graphene have potential uses in everything from batteries to biomedical devices. Researchers at Brown are looking ahead to assess the long-term environmental and human health impacts of these novel materials.
"These new atomically thin solids are being incorporated into a wide range of new technologies," said Robert Hurt, a professor of engineering. "The kind of interdisciplinary work being done at Brown to understand their potential environmental and human health effects is essential to ensure their safe development and commercialization."
Using materials from the Hurt group, Agnes Kane, a professor of pathology and laboratory medicine, showed that graphene's ultra-thin edges can cut into and damage human cells. Since then, they have worked to develop new models to better understand how these materials interact with human tissue. Most recently, Hurt, Kane and colleagues came up with a framework for categorizing hundreds of 2-D nanomaterials based on their biodegradation potential.
"Understanding the stability and biodegradation of these materials helps map them onto the right applications," Hurt said, "and also greatly influences their potential health effects and how risks should be assessed."
Hurt's nano-safety work is one of the major research themes of Brown's Superfund Research Program, a collaboration supported by a $10.8 million grant from the National Institute of Environmental Health Sciences that studies how toxicant exposures affect health and how those exposures might be contained or eliminated.
One Superfund project led by Kim Boekelheide, a professor of pathology and laboratory medicine, has developed new insights into physiological effects of toxicant exposure on the male reproductive system. Eric Suuberg, a professor of engineering, is studying how vapors from toxic material releases can re-emerge from the soil, entering into buildings built at or near the polluted sites — and why it is hard to predict the level of exposure that inhabitants of these buildings may suffer. In addition to their nano-safety work, Hurt's group is using nanomaterials to develop smart fabrics for breathable protective clothing that prevents human exposure to toxic chemicals.
Most recently, the Superfund group has been studying per- and polyfluoroalkyl substances, better known as PFAS. The chemicals, used to make everything from food packaging to fire-fighting foams, have been called "forever chemicals" because they simply do not degrade naturally. Nationwide, they have started turning up in water supplies and have been linked to cancer as well as developmental problems in children.
Superfund-related research led by Scott Frickel, a professor of sociology and environmental science, used the locations of old industrial sites to identify water supplies in Rhode Island at risk for PFAS contamination. Brown researchers then partnered with the Rhode Island Department of Health to test many of those water systems.
Research by Joseph Braun, an associate professor of epidemiology, has generated new insights on the health effects of ingesting these compounds, showing the PFAS exposure is associated with increased body fat in children. Suuberg is researching the activated carbon used to remove PFAS from water, working to figure out which kinds work best and why. Kurt Pennell, who joined the engineering faculty last year, is working on strategies to remove PFAS groundwater contamination by collecting the compounds and then destroying them.
"You form a barrier in the soil," Pennell said. "The contaminated groundwater flows through, concentrates the chemicals you want removed, then the clean water runs past. The ultimate goal is to concentrate these materials in the barriers and then react them away, and we're working with some engineered nanomaterials to do that."
The Superfund Research Program's ability to look at PFAS from a number of different perspectives — from the epidemiological research needed to understand its health effects to the chemistry and material science needed to remediate it — puts Brown in a leadership role in PFAS research, Hurt said.
"This is a major area of emerging research nationwide, and Brown is right at the center of it," he said.
Indeed, the deep expertise and collaborative research approach at the School of Engineering has positioned Brown right at the center of a wealth of energy and environmental research.
-Kevin Stacey